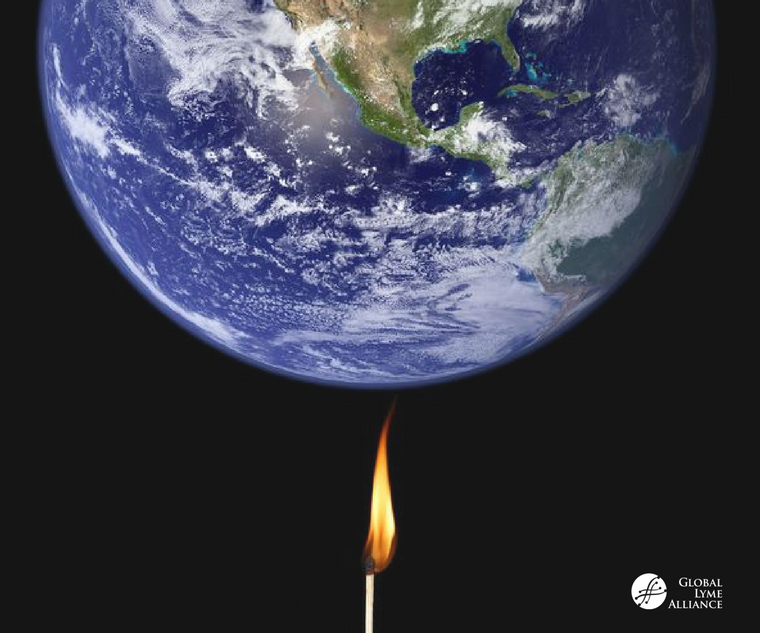
Global warming is contributing to the spread of human pathogens. Ticks and mosquitoes carrying vectorborne pathogens are increasing in population and colonizing new areas. As conditions change, new virulent pathogens are emerging
According to NASA scientists, worldwide temperatures over the past three years have been the hottest on record. In addition, average global surface temperatures have risen by 0.7°C (1.3°F) over the last 100 years. Temperatures are rising due to human activities such as the burning of fossil fuels, leading to an atmospheric accumulation of greenhouse gases (Collins et al. 2007; Karl and Trenbeth 2003). These temperature increases, along with the drought and flooding associated with global warming may be contributing to the spread of human pathogens and increased disease (Epstein 2000; EPA 2016). Warming may be causing 150,000 deaths a year when all health effects such as food poisoning, heart attacks, and heat strokes are considered. Chronic health problems associated with warming may affect millions (Patz et al. 2005). Though plant pathogens are also increasing, this article emphasizes warming effects on human and animal pathogens, especially vectorborne pathogens. Human Pathogens Pathogens for human diseases such as malaria, Lyme disease, tick-borne encephalitis, yellow fever, and dengue have increased in incidence or geographic range in recent decades (Harvell et al. 2002; Patz et al. 2005). These pathogens are carried by ticks and mosquitoes that are encouraged by warmth and moisture. For instance, the Lyme disease tick, Ixodes scapularis, thrives at temperatures above 45°F and humidities greater than 85%. Aedes aegypti mosquitoes that carry dengue and Zika virus thrive at temperatures above 50°F. Cold weather can kill these pests, but warmer and shorter winters can generate larger populations of infected mosquitoes and ticks. The overall effect is complicated by land use, worldwide travel, adaptations of vectors and pathogens, pest management and other factors (Beard et al. 2016; EPA 2016; Ogden and Lindsay 2016; Ogden et al. 2014; Kovats et al. 2001). Warmer temperatures increase mosquito reproduction and biting activity, and pathogens inside the mosquitoes mature faster. For instance, transmission of malaria requires temperatures greater In This Issue Global Warming 1 Conference Notes 8 Calendar 12 than 16°C (60.8°F), and a 5°C (9°F) increase in temperature doubles the growth rate of the falciparum protozoa that causes malaria. Since 1990 small outbreaks of malaria have occurred in Texas, Georgia, Florida, Michigan, New Jersey, New York and Toronto (Epstein 2001; Epstein 2000; Gil 1920). Warming, Droughts and Flooding Extreme weather conditions of drought and flooding caused by global warming provide breeding areas for mosquito larvae (Epstein 2000; Epstein 2005). Mosquitoes can breed after heavy rains, but also during droughts in containers used to store water. The result is a resurgence of diseases such as chikungunya fever (Epstein 2007), and expansion of pathogens such as West Nile and Zika viruses into new areas (Epstein 2000; Hayes 2009; Quarles 2016). For instance, dengue virus, West Nile virus, chikungunya virus, and Zika virus have appeared or expanded their range in the U.S. over the last 20 years (Chan et al. 2016). West Nile fever arrived in New York City in 1999. The first cases were likely travel related, but Culex spp. mosquitoes were there to spread the pathogen through populations of birds and people (Epstein 2000). West Nile fever also has invaded Canada, with confirmed cases numbering about one-fifth those of the U.S. Culex tarsalis mosquitoes are ranging further north than ever before, and epidemics surge with warm winters (Kulkarni et al. 2015). West Nile fever got its start in the U.S. when a mild winter led to large populations of mosquitoes early in the season. A subsequent drought forced Culex spp. mosquitoes that carry the pathogen into close contact with bird populations, amplifying and spreading the disease (Epstein 2001). Shrinking water holes meant that birds and mosquitoes aggregated in the same areas. Populations of predators such as dragonflies that prey on mosquitoes were reduced by drought (Epstein 2001; Epstein 2000). Aedes spp. Mosquitoes The first cases of dengue hemorrhagic fever in the U.S. were seen in Texas late in 2005 (Sci. News 2006). Chikungunya cases appeared in Texas and Florida in 2014, and Zika virus, the most dramatic new mosquito pathogen, arrived in Florida and Texas in 2016 (Epstein 2007; Chan et al. 2016; Quarles 2016). Dengue, chikungunya, and Zika viruses are all spread by Aedes spp. mosquitoes, primarily Aedes aegypti, but also Ae. albopictus and Ae. africanus (Chan et al. 2016). Areas covered by vector-borne epidemics of these viruses can be no larger than the ranges of the mosquitoes that carry them. Travel related cases cannot be sustained without effective local mosquito populations (Kraemer et al. 2015). But key vectors such as Aedes aegypti and Aedes albopictus have moved into new locations. These two species now cover the widest area ever recorded. Though they are spread by travel and trade, the most critical factor in their spread and survival is temperature (Kraemer et al. 2015; Liu-Helmersson et al. 2014). And computer models show that the ranges of these two species in the U.S. are likely to expand (Campbell et al. 2015). Not only can Aedes spp. move into warming areas that favor them, they can also adapt quickly to new conditions. Aedes japonicus actually prefers cool temperatures but can make an evolutionary adaptation to a warmer climate in less than 10 years (Egizi et al. 2015). Rising Seas Mosquitoes generally respond to rising temperatures, but rising sea levels may also be a factor. Rising sea levels encourage mosquitoes that breed in brackish or salty water. More than half the world’s population lives within 60 km (36 mi) of the coastline. This population will be at increased risk from mosquitoes such as Aedes taeniorhyncus, Culex tarsalis, and Anopheles albimanus. Culex mosquitoes can carry West Nile virus, and Anopheles mosquitoes can vector malaria (Ramasamy and Surendran 2011). More Pests, More Pathogens Greater pest numbers mean larger numbers of pathogens. Old pathogens are spreading to new areas, and new pathogens are appearing that may be better adapted to the environment (Dobson and Foufopoulos 2001). Global warming may also be encouraging more virulent strains of the microbes, such as the case for the Lyme disease pathogen (Ostfeld and Brunner 2015; Gatewood et al. 2009; Ogden et al. 2008) and the Zika virus (Chan et al. 2016). For instance, less than 20 human cases of Zika virus infection had been reported worldwide before 2007. Increased populations of Aedes aegypti encouraged by global warming and increased rainfall, and genetic changes in the Zika virus RNA genome may have both contributed to the explosive outbreaks of the Asian strain of Zika in Latin America in 2015, and its arrival in the U.S. in 2016 (Chan et al. 2016; Quarles 2016). Tick-borne DiseasesConfirmed Lyme disease cases in the U.S. have doubled in the last 20 years. Both the areas affected and the number of cases are increasing.Confirmed Lyme disease cases in the U.S. have doubled in the last 20 years. Both the areas affected and the number of cases are increasing. The EPA has estimated that there are 300,000 new cases of Lyme disease each year in the U.S. (EPA 2016). Lyme disease is caused by a spirochete called Borrelia burgdorferi, that is carried by Ixodes scapularis in the East and Ixodes pacificus in the West. Spirochetes are corkscrew shaped bacteria that cause a number of diseases including relapsing fever, leptospirosis, syphilis, and others. Lyme disease, like syphilis, can cause longterm chronic problems involving the nervous system. B. burgdorferi shows a lot of genetic variability, and some genotypes may be harder to treat than others (Sonenshine 1993; Burgdorfer 1993; Quarles 2000). Due to warming, Lyme disease ticks are spreading into new areas, such as the southern regions of Canada (Leighton et al. 2012; Brownstein et al. 2005). Confirmed Lyme disease cases in Canada have increased more than 10-fold since 2005 (Kulkarni et al. 2015). Ticks in Motion The major pathogenic tick in the Northeast is Ixodes scapularis. It carries Lyme disease and a number of other pathogens. The major tick in the Southeast is the lone star tick, Amblyomma americanum, which carries Ehrlichiosis and other diseases. Ticks in the Southern U.S. such as Amblyomma spp. are spreading northward and westward, carrying new pathogens. And a new tick, Ixodes affinis has been found in North Carolina. Monitoring shows about one-third of I. affinis are carrying B. burgdorferi (Stromdahl and Hickling 2012). Tick populations associated with warming have contributed to increased tickborne encephalitis in Sweden, as infected ticks move northward (Lindgren and Gustafson 2001). Lethal tickborne Crimean Congo hemorrhagic fever appeared for the first time in Western Europe in 2016. It is usually found in colder regions of Eastern Europe and the Soviet Union (McNeil 2016). Phenology and Ecology Important Global warming may be encouraging the spread of pathogens, not just through increased temperatures, but also through phenology and changes in ecology. Ecology is important because deer spread the Lyme disease tick, and white-footed mice are a major reservoir for the infection. Changes in the distribution of these populations can change the risk for Lyme disease (Bosler 1993). Phenology and the timing of the infection is also important. For instance, the Lyme disease tick, Ixodes scapularis, has egg, larval, nymphal and adult stages on a two-year cycle. Adults lay eggs in soil in June or early July that hatch into larvae starting in July. Larvae start feeding in July, and most have molted into nymphs by the following spring. If the larvae feed on an infected host, then the nymphal stage will also be infected (Bosler 1993). Nymphs spread the infection. Infected nymphs inoculate vertebrate hosts with the pathogen starting in the spring. Early spring activity of nymphs due to shorter and warmer winters makes it possible for them to inoculate a larger percentage of the white-footed mice populations before uninfected larval ticks begin feeding in July. Larger numbers of infected mice increase the numbers of infected larvae. So the overall effect of global warming on Ixodes scapularis is increased numbers of ticks carrying the pathogen (Levi et al. 2015). The situation is somewhat different in California, where the Ixodes pacificus tick is on a three-year cycle, and spends most of its time off host. This tick is susceptible to periods of drought and low rainfall. Compared to Northern California, populations in Southern California are lower due to lower rainfall, and the Lyme disease risk is less, despite the fact that Southern California has warmer winters. This kind of regional variation is superimposed on the overall effects due to global warming (MacDonald and Briggs 2016). Changes in Animal Migrations Animals can be important carriers of human pathogens. Birds carry West Nile virus, white-footed mice carry the pathogen of Lyme disease. Birds can carry infected ticks such as Ixodes scapularis and scatter them along flight paths. Changes in migration patterns caused by global warming and development may lead to concentrations of pathogens in new areas (Altizer et al. 2011; Hofmeister et al. 2012). New Tickborne Pathogens As ticks move into new areas, they pick up new pathogens or the old ones adapt to new conditions. From 2010 to 2016, five new human tickborne pathogens were identified in the U.S. These include Borrelia mayonii (2016), Bourbon virus (2014), Heartland virus (2012), Borrelia miyamotoi (2011), and the microbe for 364D rickettsiosis (2010) (Pritt et al. 2016; Krause et al. 2014; Shapiro et al. 2010). The accelerated pace of discovery is astounding, since only six new pathogens, including those for Lyme disease, Babesiosis and Ehrlichiosis were found in the 32 year period from 1969 to 2002, doubling at that time the number of known tickborne pathogens (Stromdahl and Hickling 2012). Tick Coinfections A bite that transmits Lyme may also transmit other pathogens, resulting in clinical cases of multiple infections. For instance, the Lyme disease tick Ixodes scapularis, carries Borrelia burgdorferi for Lyme disease; Anaplasma phagocytophylium that causes anaplasmosis; Babesia microti that causes babesiosis; and Powassen virus that causes Powassen encephalitis (Stromdahl and Hickling 2012). There are often co-infections with mixed Borrelia such as B. miyamotoi and B. burgdorferi. In the Northeast the human infection rate with B. burgdorferi is about twice that of B. miyamotoi (Krause et al. 2012). In the San Francisco Bay Area, I. pacificus ticks infected with B. burgdorferi are about as abundant as those with B. miyamotoi (Salkeld et al. 2014). The lone star tick, Amblyomma americanum carries Ehrlichia spp. organisms that cause Ehrlichiosis; Francisella tularensis that causes tularemia; Rickettsia rickettsii that causes spotted fever rickettsiosis; and weird diseases such as southern tick-associated rash illness (STARI). Bites can cause multiple diseases and immune system problems (Stomdahl and Hickling 2012). Global Warming and Fungal Diseases Vectorborne pathogens are usually viruses or bacteria. But new fungal diseases of humans and wildlife are appearing. Fungal pathogens are becoming more virulent, and medical databases show the relative proportion of emerging infectious diseases in animals caused by fungi tripled from 1995 to 2010 (Fisher et al. 2012). Warming may be encouraging growth and spread of fungi, and animal immune systems may also be depressed both by warming and pesticides (Altizer et al. 2013; Mason et al. 2013). For instance, global warming may be contributing to amphibian decline. Though chytrid fungus, Batrachochytrium dendrobatidis, has been around since 1928, it was 1998 before it was identified as a lethal pathogen of amphibians (Quarles 2015). Growth is associated with warmer summers, and cloud cover associated with increased water evaporation (Pounds et al. 2006). And white nose fungus caused by Geomyces destructans, has killed millions of bats in the U.S. since 2006 (Quarles 2013). Fungi Attacking Humans There has been a resurgence of human fungal diseases caused by Aspergillus, Candida and others. Part of the resurgence is due to HIV and medical procedures such as chemotherapy and organ transplant that depress immune function. Deaths from these fungal diseases worldwide now exceed those of malaria and tuberculosis combined, and these fungal diseases are often resistant to treatment (Berweij et al. 2009). For instance, a new lethal antibiotic resistant human fungus, Candida auris, appeared in hospitals in 2009 (Brown et al. 2012). But part of the resurgence of fungal diseases in humans may be due to global warming. Cryptococcus gattii is usually a pathogen in tropical and subtropical areas, but in 2001 a lethal strain appeared on Vancouver Island in Canada. And global warming may have contributed to the eightfold increase in U.S. valley fever cases caused by Coccidioides immitis between 1999 and 2011 (Frazer 2013). Fungi Attacking Humans There has been a resurgence of human fungal diseases caused by Aspergillus, Candida and others. Part of the resurgence is due to HIV and medical procedures such as chemotherapy and organ transplant that depress immune function. Deaths from these fungal diseases worldwide now exceed those of malaria and tuberculosis combined, and these fungal diseases are often resistant to treatment (Berweij et al. 2009). For instance, a new lethal antibiotic-resistant human fungus, Candida auris, appeared in hospitals in 2009 (Brown et al. 2012). But part of the resurgence of fungal diseases in humans may be due to global warming. Cryptococcus gattii is usually a pathogen in tropical and subtropical areas, but in 2001 a lethal strain appeared on Vancouver Island in Canada. And global warming may have contributed to the eightfold increase in U.S. valley fever cases caused by Coccidioides immitis between 1999 and 2011 (Frazer 2013). One theory about the rise of pathogenic fungi in mammals is that global warming is causing fungi to adapt to higher temperatures. As a result, the gap between mammalian temperatures and optimum temperatures for fungi is becoming smaller (Casadevall 2012; Garcia-Solache et al. 2010). Runoff and Ocean Warming Ocean warming and acidification plus nutrient loaded runoff from flooding is leading to increased algae blooms and shellfish poisoning from domoic acid and other toxins. Poisonous crabs and shellfish are having an impact on the fishing industry. Warming conditions are also encouraging growth of zooplankton containing the Vibrio cholerae organism, resulting in increased numbers of human cholera cases (Altizer et al. 2013; Brown et al. 2012). Increased runoff from flooding is carrying the pathogen Toxoplasma gondii from cat feces into the oceans. Dolphins and other marine animals are being killed by lethal infections of toxoplasmosis (Solomon 2013). What to do About All This? Global warming is associated with human activities such as the burning of fossil fuels, leading to a buildup of carbon dioxide in the atmosphere. Carbon dioxide exerts a greenhouse effect, causing increased global temperatures (Quarles 2007). We can reduce global warming by encouraging renewable energy such as solar electricity, wind farms, and hydroelectric power. We can drive fuel efficient cars. Since about 15% of the greenhouse emissions are due to farming, we can encourage organic agriculture. Each acre of organic production takes about 3,500 lbs (1590 kg) of carbon dioxide from the air each year. About 20% of CO2 emissions is caused by tropical deforestation. So planting trees can help reduce the problem (Hepperly 2007; Rosenzweig and Hillel 1995). Mosquito Management To protect ourselves from vector-borne pathogens, we can use IPM methods such as habitat management, repellents, reduction of breeding sources, and mosquito larval control. Mosquito adulticides are likely to have effects on nontarget organisms such as bees (Olkowski et al. 1991; Quarles 2011; Quarles 2001; Olkowski 2001). Mosquito repellents such as oil of lemon eucalyptus, picaridin, and deet can protect against mosquito bites (Quarles 2009). Mosquitoes such as Aedes spp. that carry Zika virus, yellow fever, encephalitis, and dengue breed in containers and bite in the daytime. Removing containers of water around dwellings, and treatment of other water sources with Bacillus thuringiensis israelensis (BTI) and IGRs can help control the problem (Olkowski et al. 1991). Tick Management Ticks are vulnerable to desiccation. Habitat management such as opening up tree canopies for sunlight, removal of leaf litter, mowing lawns can discourage them. Strategies such as bait stations to remove ticks from deer and mice are useful (Olkowski et al. 1991; Quarles 2010). Wearing clothing treated with tick repellents reduces the danger (Benelli et al. 2016; Jordan et al. 2012). As a last resort, areas around dwellings can be treated with reduced risk pesticides (Quarles 2010)(see below). Tick Biocontrol The fungus Metarhizium anisopliae is pathogenic to all stages of the ticks and has much potential as a biocontrol agent. Field tests on 100 m2 plots averaging 10 ticks per plot showed M. anisopliae killed about 53% of the ticks (Benjamin et al. 2002; Quarles 2003). Application of M. anisopliae when nymphal ticks were active led to an 87-96% reduction, but populations bounced back, and after 5 weeks reduction was 53-74% (Bharadwaj and Stafford 2010). Other field tests showed about a 56% tick reduction on lawns treated with M. anisopliae or Beauveria bassiana (Stafford and Allan 2010). Least-Toxic Tick Control A number of botanicals can be useful in tick management. The compound noonkatone from cedar oil is one of the most effective. Ixodes scapularis tick reductions of about 96% for 42 days were seen in New Jersey. However, commercial development has been slow (Jordan et al. 2011). Application of the commercial essential oil formulation IC2 to oak pine forests in Maine was just as effective as maximum label applications of bifenthrin for control of blacklegged tick, I. scapularis. Tick populations were controlled for 6-9 months by the essential oil. Bees were not affected, but some non-target insects showed decline for about 3 weeks. Bifenthrin protection lasted 12-16 months and had more of an impact on non-target populations (Elias et al. 2013; Rand et al. 2010). Garlic has also been used with moderate success to control ticks. Application of Mosquito Barrier (0.2 g AI/m2) to residential properties in Connecticut led to about 50% suppression of nymphal I. scapularis ticks for about two weeks (Bharadwaj et al. 2015). Conclusion Global warming is contributing to the spread of human pathogens. Ticks and mosquitoes carrying vectorborne pathogens are increasing in population and colonizing new areas. As conditions change, new virulent pathogens are emerging. The trends seen now will likely worsen with the higher global temperatures expected by the end of the century. We can fight global warming with renewable energy, organic agriculture, and aggressive tree planting programs. These approaches will help reduce warming caused by carbon dioxide greenhouse gases. Vectorborne pathogens can be reduced by IPM methods such as exclusion, habitat management, repellents and least toxic pesticides. Acknowledgement The author would like to thank Erin Berg for help with research on this article. William Quarles, Ph.D., is an IPM Specialist, Executive Director of the Bio-Integral Resource Center (BIRC), and Managing Editor of the IPM Practitioner. He can be reached by email, birc@igc.org *Opinions expressed by contributors are their own. References Altizer, S., R. Bartel and B.A. Han. 2011. Animal migration and infectious disease risk. Science 331:296-302. Altizer, S., R.S. Ostfeld, P.T.J. Johnson et al. 2013. Climate change and infectious diseases: from evidence to a predictive framework. Science 341:514-519. Update Beard, C.B., R.J. Eisen, C.M. Barker et al. 2016. Vectorborne Diseases. In: Crimmins, A.J., J. Balbus, J.L. Gamble et al. The Impacts of Climate Change on Human Health in the U.S., U.S. Global Change Research Program. Washington, DC. 312 pp. Benelli, G., R. Pavela, A. Canale et al. 2016. Tick repellents and acaricides of botanical origin: a green roadmap to control tick-borne diseases? Parasitol. Res. 115:2545-2560. Benjamin, M.A., E. Zhioua and R.S. Osfeld. 2002. Laboratory and field evaluation of the entomopathogenic fungus Metarhizium anisopliae for controlling questing adult Ixodes scapularis. J. Med. Entomol. 39(5):723-728. Berweij, P.E., E. Snelders, G.H.J. Kema. 2009. Azole resistance in Aspergillus fumigatus: a side-effect of environmental fungicide use? Lancet Infect. Dis. 9:789-795. Bharadwaj, A. and K.C. Stafford, III. 2010. Evaluation of Metarhizium anisopliae strain F52 for control of Ixodes scapularis. J. Med. Entomol. 47(5):862-867. Bharadwaj, A., L.E. Hayes, and K.C. Stafford, III. 2015. Effectiveness of garlic for control of Ixodes scapularis on residential properties in western Connecticut. J. Med. Entomol. 52(4):722-725. Bosler, E.M. 1993. Tick vectors and hosts. In: Coyle, pp. 18-26. Brown, G.D., D.W. Denning, N.A.R. Gow et al. 2012. Hidden killers: human fungal infections. Sci. Transl. Med. 4(165):1-7. Brownstein, J.S., T.R. Holford and D. Fish. 2005. Effect of climate change on Lyme disease risk in North America. EcoHealth 2(1):38-46. Burgdorfer, W. 1993. Discovery of Borrelia burgdorferi. In: Coyle, pp. 3-7. Campbell, L.P., C. Luther, D. M.-Llanes et al. 2015. Climate change influences on global distributions of dengue and chikungunya virus vectors. Phil. Trans. Royal Soc. B. 370:20140135. Casadevall, A. 2012. Fungi and the rise of mammals. PLoS Pathog. 8(8):e1002808. Chan, J.F.W., G.K.Y. Choi, C.C.Y. Yip et al. 2016. Zika fever and congenital Zika syndrome: an unexpected emerging arboviral disease. J. Infect. 507-524.
